The Ultimate Cheat Sheet For SPACE – UPSC Prelims 2025
Last Updated
25th April, 2025
Date Published
25th April, 2025
Share This Post With Someone
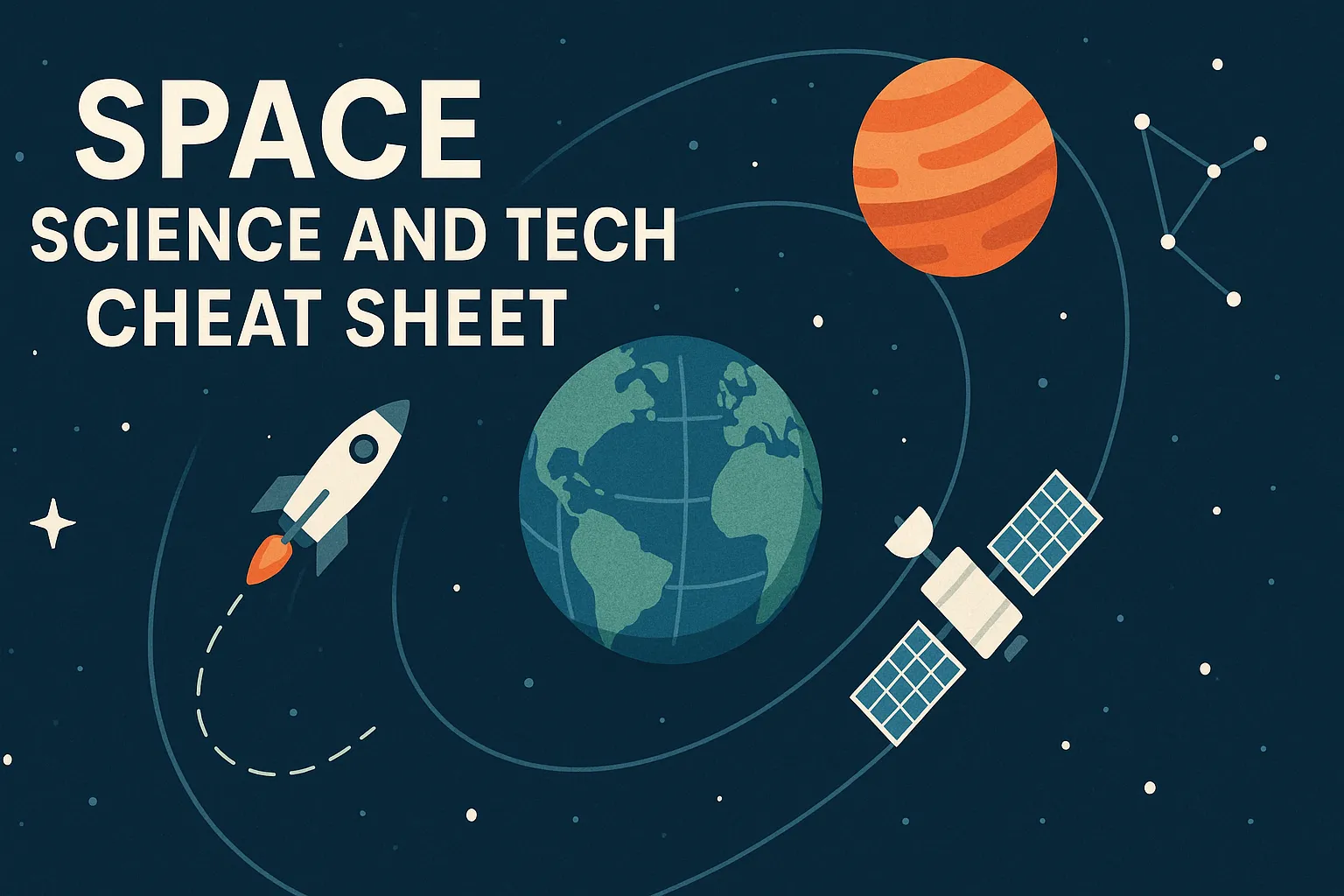
Want To Print The Cheat Sheet & Prepare On The Go?
Introduction to Space
Space begins approximately 100 kilometers (60 miles) above Earth's surface, marking the boundary between our planet's atmosphere and outer space. This region represents the vast cosmos beyond our planet, containing numerous celestial objects and phenomena governed by fundamental physical forces, particularly gravity.
Cosmological Phenomena
Dark Energy
Dark energy is a mysterious force driving the accelerating expansion of our universe, contradicting the expectation that gravity would slow this expansion over time. It has been dubbed "dark" because of its invisible nature, yet it appears to exert significant influence on cosmic scales, counteracting gravitational forces that would otherwise pull matter together. The discovery of accelerating cosmic expansion rather than deceleration represents one of the most profound cosmic mysteries modern astrophysics seeks to solve.
Dark Matter
Dark matter consists of particles that lack electromagnetic charge, meaning they don't interact through electromagnetic forces. This unique property explains why dark matter:
- Does not emit light (an electromagnetic phenomenon)
- Remains invisible to direct observation
- Still possesses mass like normal matter
- Interacts through gravitational forces
These characteristics make dark matter detectable only through its gravitational effects on visible matter, despite comprising a significant portion of the universe's mass.
Stellar Objects and Life Cycles
Stars and Their Formation
- Stars represent the fundamental luminous objects in our universe, with varied sizes, temperatures, and lifespans depending on their initial mass. They form from condensing clouds of hydrogen and helium, with gravity pulling this material together until nuclear fusion begins in their cores, releasing tremendous energy in the form of heat and light.
Chandrasekhar Limit
- The Chandrasekhar Limit states that no white dwarf (a collapsed, degenerate star) can exceed approximately 1.4 times the Sun's mass. This fundamental astrophysical constraint explains why stars above this threshold cannot end their lives as white dwarfs—instead, they face more extreme fates such as becoming neutron stars or black holes. The limit, named after physicist Subrahmanyan Chandrasekhar, represents a critical boundary in stellar evolution.
Black Holes
Black holes are regions where gravity is so intense that nothing, not even light, can escape once passing their event horizon. Key characteristics include:
- Extraordinarily strong gravitational fields
- Complete invisibility (though detectable through their effects)
- Formation typically from collapsed massive stars
Supermassive Black Holes
These cosmic giants possess masses ranging from millions to billions of solar masses. They reside at the centers of most large galaxies, including our Milky Way, and play crucial roles in galactic evolution and structure. Their formation mechanisms remain an active area of research in modern astrophysics.
Eddington Limit
The Eddington limit governs the maximum rate at which a black hole can consume matter. This theoretical constraint balances the inward pull of gravity against the outward push of radiation pressure, effectively regulating how quickly black holes can grow through accretion. When black holes exceed this feeding rate, they produce powerful outflows that can affect surrounding star formation.
White Holes
White holes represent theoretical cosmic entities functioning opposite to black holes - while nothing escapes black holes, nothing can enter white holes. Physicists describe them as black holes' "time reversals," with properties that mirror their better-known counterparts. Though mathematically predicted by some solutions to Einstein's field equations, no observational evidence currently confirms their existence.
Quasars
Quasars (quasi-stellar radio sources) are extraordinarily luminous objects found in distant galaxies that emit powerful jets detectable at radio frequencies. They exclusively exist in galaxies hosting supermassive black holes, which power their bright accretion discs. These objects represent some of the universe's most energetic phenomena, often outshining entire galaxies despite originating from relatively compact regions.
The Sun
Composition and Structure
The Sun, the largest object in our solar system, consists primarily of hydrogen and helium in a hot, glowing state. Its structure includes several distinct regions with varying temperatures and physical properties. Three primary internal layers comprise:
- Core: Nuclear fusion center
- Radiative zone: Energy transfer through radiation
- Convective zone: Energy transfer through convection
Solar Atmosphere Layers
Photosphere
The photosphere represents the deepest visible layer of the Sun, exhibiting a temperature gradient from approximately 6500K at its base to 4000K at its upper boundary. This region is what we perceive as the Sun's surface, though the Sun lacks a solid surface in the conventional sense.
Chromosphere
The chromosphere extends above the photosphere with temperatures ranging from about 4000K at its lower boundary to 8000K at its upper edge. Intriguingly, unlike most thermal systems, the chromosphere's temperature increases with distance from the Sun, contradicting expected thermal behavior.
Transition Region
This extremely narrow layer (approximately 100 km/60 miles thick) forms the boundary between the chromosphere and corona, featuring a dramatic temperature increase from about 8000K to approximately 500,000K. This abrupt thermal gradient represents one of solar physics' most puzzling features.
Corona
The corona constitutes the Sun's outermost atmospheric layer, with temperatures exceeding 500,000K. Despite these extreme temperatures, the corona appears dim relative to the photosphere due to its low density. The corona lacks a defined upper boundary, effectively extending into interplanetary space and transitioning into the solar wind.
Solar Phenomena
Solar Wind
The solar wind comprises a continuous stream of charged particles (primarily electrons and protons) flowing outward from the Sun. This plasma originates in the corona, where extreme temperatures accelerate particles to velocities sufficient to escape the Sun's gravitational pull. The solar wind extends the Sun's influence throughout the solar system, interacting with planetary environments.
Sunspots
Sunspots appear as dark areas on the Sun's photosphere. Their relatively cool temperatures (about 3000-4500K compared to the surrounding 5800K photosphere) result from intense magnetic field concentrations that inhibit heat transport from deeper layers. These magnetic anomalies serve as indicators of solar activity and often correlate with other solar phenomena.
Solar Flares
Solar flares represent sudden, explosive releases of energy occurring when magnetic field lines near sunspots tangle, cross, and reconfigure. These events release enormous radiation bursts across the electromagnetic spectrum and can significantly impact Earth's technological systems by:
- Disrupting satellite operations
- Interfering with radio transmissions
- Potentially damaging power grid transformers during severe geomagnetic storms
Coronal Mass Ejections (CMEs)
CMEs are massive eruptions of radiation and particles from the Sun's corona that explode into space at extremely high velocities. These events occur when magnetic field lines suddenly reorganize, releasing tremendous energy and propelling solar material outward. Upon reaching Earth, CMEs can trigger geomagnetic storms and spectacular auroral displays.
Solar-Terrestrial Interactions
Auroras
Auroras manifest as natural light displays in high-latitude regions (Arctic and Antarctic). These colorful atmospheric phenomena result from charged particles (mainly electrons and protons) entering Earth's atmosphere from space, causing ionization and excitation of atmospheric gases that subsequently emit visible light. The auroras' characteristic colors correspond to different atmospheric gases and excitation energies.
Magnetosphere Protection
Earth's magnetosphere shields our planet from the solar wind by deflecting incoming charged particles. This protective magnetic bubble prevents harmful radiation from reaching Earth's surface, enabling life to flourish. The magnetopause marks the boundary between Earth's magnetic domain and the solar wind, though partial magnetic reconnection allows some particles to penetrate this barrier.
Planets and Dwarf Planets
Definition Criteria
In 2006, the International Astronomical Union (IAU) established three criteria for classifying an object as a planet:
- It must orbit a fully-fledged star
- It must possess sufficient gravity to maintain a spherical shape
- It must have cleared its orbital neighborhood of other objects
This final criterion differentiates planets from dwarf planets, leading to the reclassification of several previously recognized planets, most famously Pluto.
Dwarf Planets
Dwarf planets orbit the Sun directly and have sufficient mass for self-gravity to maintain a roughly spherical shape but have not cleared their orbital neighborhoods of other objects. The IAU currently recognizes five named dwarf planets: Ceres, Pluto, Eris, Haumea, and Makemake. All except Ceres (located in the asteroid belt) are classified as Plutoids, dwelling in the outer solar system beyond Neptune.
Minor Celestial Bodies
Asteroids and the Asteroid Belt
Asteroids comprise rocky remnants from the solar system's formation period. Most occupy the asteroid belt between Mars and Jupiter's orbits, representing material that failed to coalesce into a planet due to Jupiter's gravitational influence. These objects range dramatically in size from tiny fragments to bodies hundreds of kilometers in diameter.
Asteroid Defense
The Asteroid Impact Deflection Assessment (AIDA) represents one planetary defense method against potentially hazardous asteroids. AIDA and related missions such as NASA's DART (Double Asteroid Redirection Test) aim to test asteroid deflection techniques, including:
- Kinetic impactors that strike asteroids to alter their trajectory
- Alternative methods such as explosive deflection
Potentially Hazardous Asteroids (PHAs) are specifically defined as objects with a minimum orbit intersection distance of 0.05 astronomical units or less and an absolute magnitude of 22.0 or less.
Comets
Comets consist of ice, dust, and rocky material that typically originate in the outer solar system. When approaching the Sun, their volatile components vaporize, creating characteristic comas and tails that can stretch for millions of kilometers. These objects provide valuable insights into the early solar system's composition.
Kuiper Belt
The Kuiper Belt extends beyond Neptune's orbit, containing numerous small bodies remaining from the solar system's formation. This region:
- Spans approximately 20 times wider than the asteroid belt
- Contains 20-200 times more mass than the asteroid belt
- Houses several dwarf planets including Pluto, Eris, Makemake, and Haumea
The Kuiper Belt contains two main object classifications: "cold" and "hot" classical objects, differentiated by their orbital characteristics and Neptune's gravitational influence on their trajectories.
Oort Cloud
The Oort Cloud forms a vast spherical shell surrounding the entire solar system at its outermost boundaries. This region serves as the primary source for long-period comets entering the inner solar system. Unlike the relatively flat Kuiper Belt, the Oort Cloud extends in all directions, potentially containing trillions of icy bodies.
Orbital Mechanics
Lagrange Points
Lagrange points represent special positions where small objects can maintain stable positions relative to two larger bodies (such as the Sun and Earth). These mathematically derived locations emerge from the "General Three-Body Problem" in celestial mechanics and include:
- Unstable points (L1, L2, L3) aligned with the two large masses
- Stable points (L4, L5) forming equilateral triangles with the large masses
These locations have significant practical applications for space-based observatories and other missions requiring minimal station-keeping.
Orbit Types
Based on Shape
- Circular Orbits: Perfect circles with eccentricity of 0
- Elliptical Orbits: Oval-shaped paths with eccentricity between 0 and 1, causing satellites to travel at varying speeds depending on their distance from Earth
Based on Inclination
Inclination measures the angle between an orbit and Earth's equatorial plane, determining which parts of Earth a satellite passes over.
Equatorial Orbits
Equatorial orbits follow Earth's equator with zero inclination. Satellites in these orbits require launch locations near the equator and prove especially useful for monitoring tropical weather patterns and providing communications services to equatorial regions. These orbits typically occupy low or medium altitudes.
Polar Orbits
Polar orbits maintain approximately 90-degree inclination to the equator, passing over both poles on each orbital pass. This configuration allows satellites to eventually observe virtually every part of Earth as the planet rotates beneath them, making these orbits ideal for mapping, surveillance, and Earth observation missions. Polar orbiting satellites typically travel north-to-south rather than west-to-east.
Geostationary Orbit (GEO)
Geostationary orbits represent specialized equatorial circular orbits where satellites:
- Maintain fixed positions relative to Earth's surface
- Complete one orbit precisely matching Earth's rotational period
- Position approximately 36,000 km above Earth's surface
- Have zero inclination (directly above the equator)
These unique characteristics make geostationary orbits invaluable for telecommunications, weather monitoring, and continuous regional surveillance. Importantly, only one geostationary orbital path exists, though it can host multiple satellites at different longitudinal positions.
Geosynchronous Orbit
Geosynchronous orbits match Earth's rotational period but have non-zero inclination. Satellites in these orbits return to the same position in the sky after one sidereal day when viewed from a fixed ground location, but their apparent position traces a figure-eight pattern due to their inclination. This orbit type offers advantages for communication networks requiring coverage of non-equatorial regions.
Sun-Synchronous Orbit (SSO)
Sun-synchronous orbits represent specialized polar orbits where satellites maintain fixed positions relative to the Sun, passing over the same Earth locations at consistent local times each day. This synchronization results from careful orbital design accounting for Earth's rotation and revolution. These orbits typically operate at 600-800 km altitudes with speeds around 7.5 km/second, making them ideal for Earth observation missions requiring consistent lighting conditions.
Orbital Regions
Low Earth Orbit (LEO)
Low Earth Orbit encompasses the region 160-2000 km above Earth's surface. Satellites here:
- Complete orbits in approximately 90-120 minutes
- Represent the most populous and accessible orbital realm
- Support communication and imaging applications
- Require less launch energy (thus lower cost)
However, LEO presents significant challenges including atmospheric drag reducing satellite lifespans and increasing space debris hazards requiring protective measures for assets like the International Space Station.
Medium Earth Orbit (MEO)
Medium Earth Orbits occupy the region between 2000 km and 35,786 km (just below GEO), with orbital periods ranging from 2-24 hours. Notable MEO examples include:
- Semi-synchronous orbit: A near-circular path approximately 20,200 km above Earth's surface with a 12-hour orbital period
- Molniya orbit: A highly elliptical orbit providing extended coverage over high-latitude regions
MEO balances the advantages and disadvantages of LEO and GEO, offering good Earth coverage with moderate latency.
Space Environment
Microgravity and Its Effects
Microgravity conditions in space create numerous unique phenomena:
- Fire burns differently due to altered convection patterns
- Crystal growth improves without gravitational distortion
- Human physiology undergoes significant changes, including cardiac weakening as the heart no longer pumps against gravity, leading to decreased red blood cell production and immunodeficiency
These effects pose substantial challenges for long-duration spaceflight and have prompted extensive research into countermeasures.
Artificial Gravity
The "Human Eccentric Rotator Device" (HERD) represents one compact solution designed to counteract weightlessness's negative physiological effects. Such technologies aim to simulate gravity through centrifugal force, potentially mitigating the health risks associated with extended microgravity exposure during long-duration space missions.
Conclusion
This compilation covers the essential astronomical concepts presented in the source material, from cosmic-scale phenomena like dark energy to the detailed classification of Earth's orbital regions. Understanding these fundamentals provides a solid foundation for appreciating both the wonders of our universe and the technical challenges of space exploration. The notes capture the intricate relationships between various celestial objects and phenomena while explaining their significance to both scientific research and practical applications in space technology.
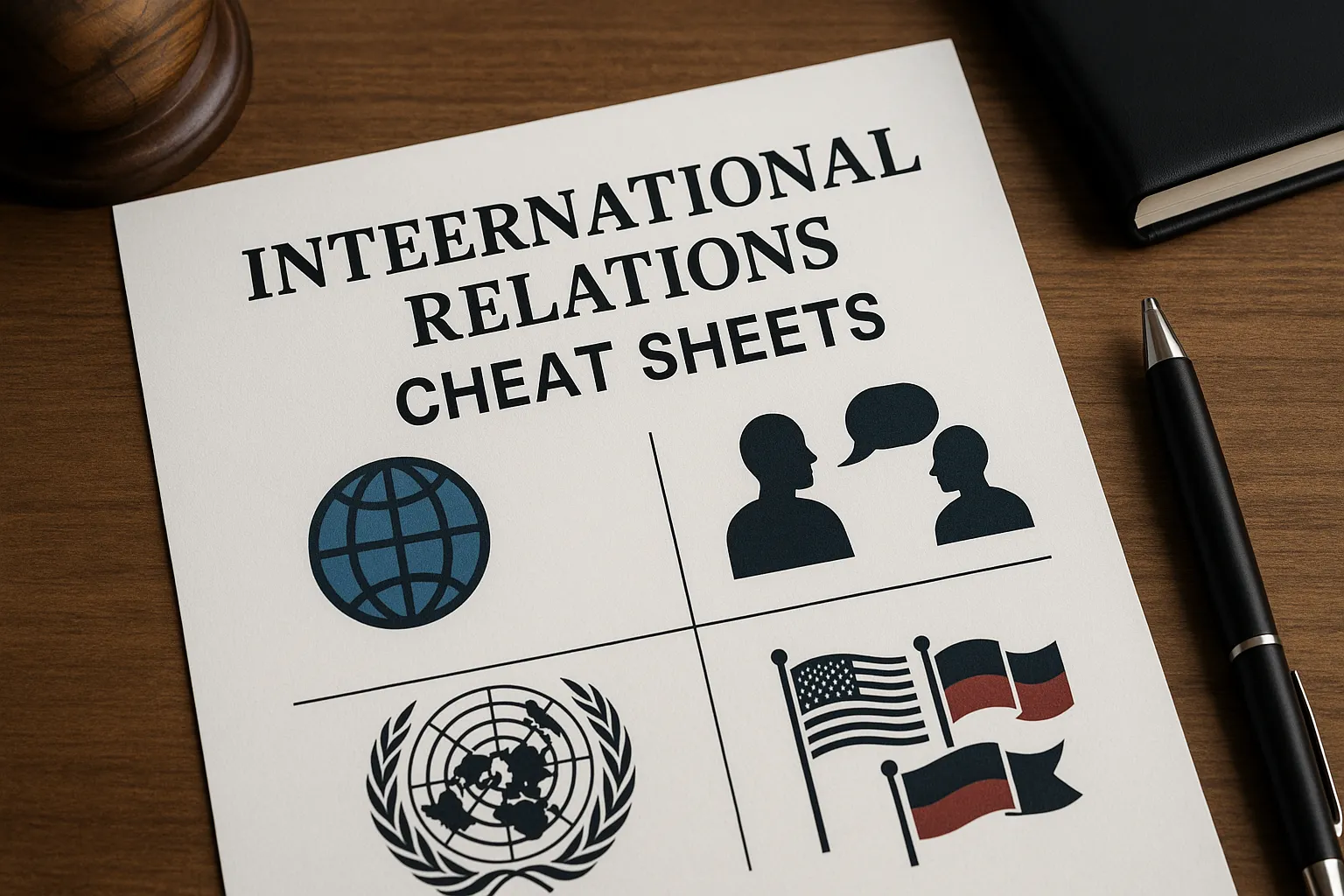
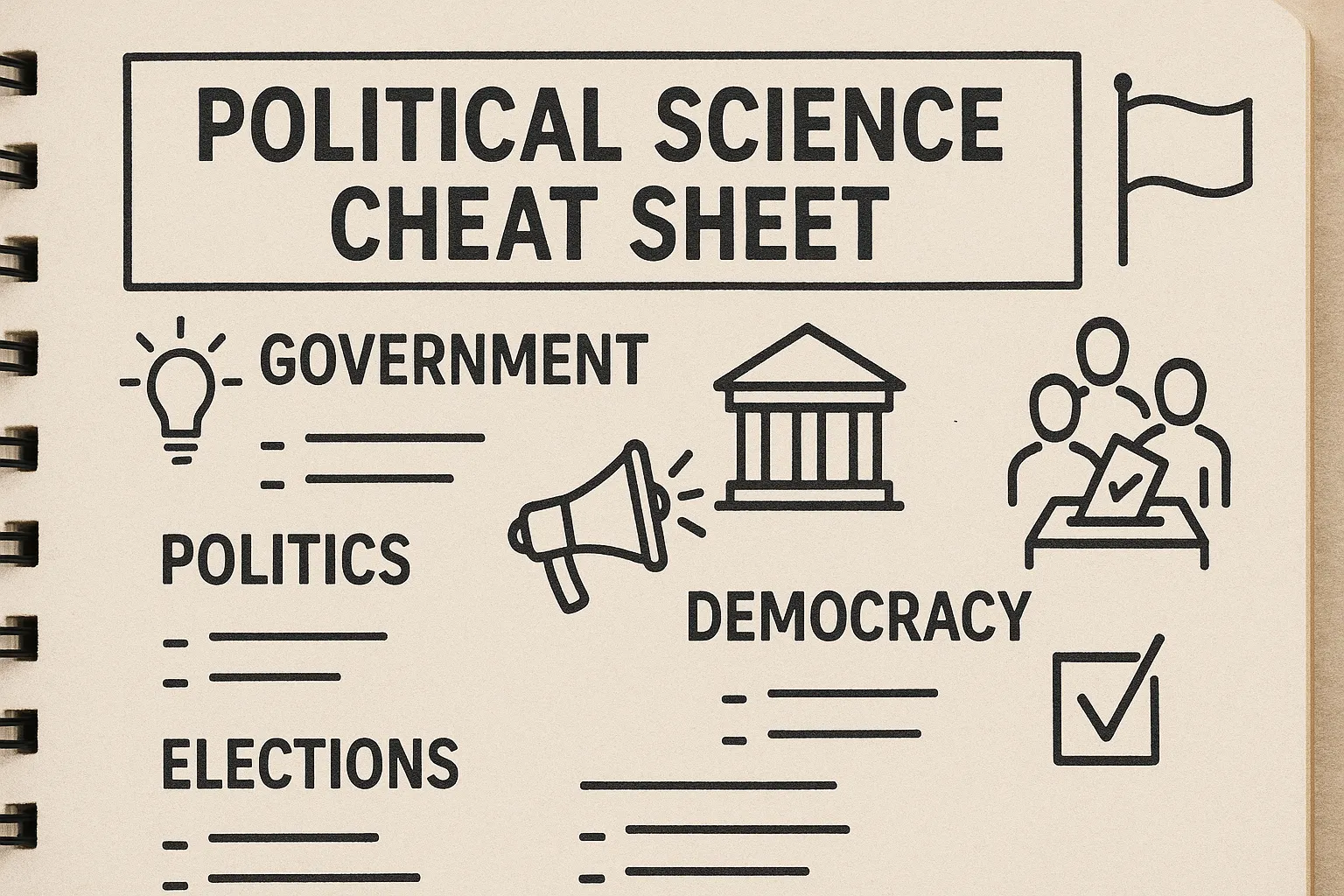